The Biology Behind CRISPR/Cas
The media has been very crispy about CRISPR. However, the actual credit should go to Cas — the endonuclease protein — rather than CRISPR…
The media has been very crispy about CRISPR. However, the actual credit should go to Cas — the endonuclease protein — rather than CRISPR, the region present in the bacterial genome.
The Covid era has taught us a great deal about immunity. When you receive a vaccine for the virus, you are creating a memory for your immune system. You are training your body’s adaptive immune system, which alone contains countless cells and numerous cell types. Now imagine an adaptive immune system within a single bacterial cell!
CRISPR, or Clustered Regularly Interspaced Short Palindromic Repeats, is a region in the bacterial genome that confers adaptive immunity to the bacteria. This region consists of regularly spaced repeats with intercalated sequences called spacers. Although there are mainly three types of CRISPR systems, I’ll be discussing the Type II system here.
These spacers are actually derived from viral DNA. When a new virus attacks bacterial cells, short sequences are excised from the viral DNA and stored between the repeats in the CRISPR region.
The CRISPR region is then transcribed into pre-crRNAs, or precursor CRISPR RNAs. These are processed and cleaved into smaller crRNAs, with each crRNA containing a spacer and a repeat. Another RNA molecule called trans-activating crRNA, or tracrRNA, is also transcribed, which forms an RNA duplex with the repeat region of the crRNA at the 3' end. These crRNAs and tracrRNA are then loaded onto endonuclease proteins called Cas (CRISPR associated proteins).
Cas, loaded with RNA, surveils the bacterial cells in search of sequences that are complementary to the spacer. Therefore, if the same virus (from which the spacer was derived and inserted into CRISPR) re-infects the bacterial cells, the viral DNA containing the spacer-complementary sequence will form an RNA-DNA hybrid with the spacer, dislodging the non-complementary strand. This results in a structure called R-Loops.
Once bound to the target DNA sequence, different domains of Cas cleave the two strands, producing a Double Strand Break (DSB), thus preventing the viral DNA from affecting the bacterial cell.
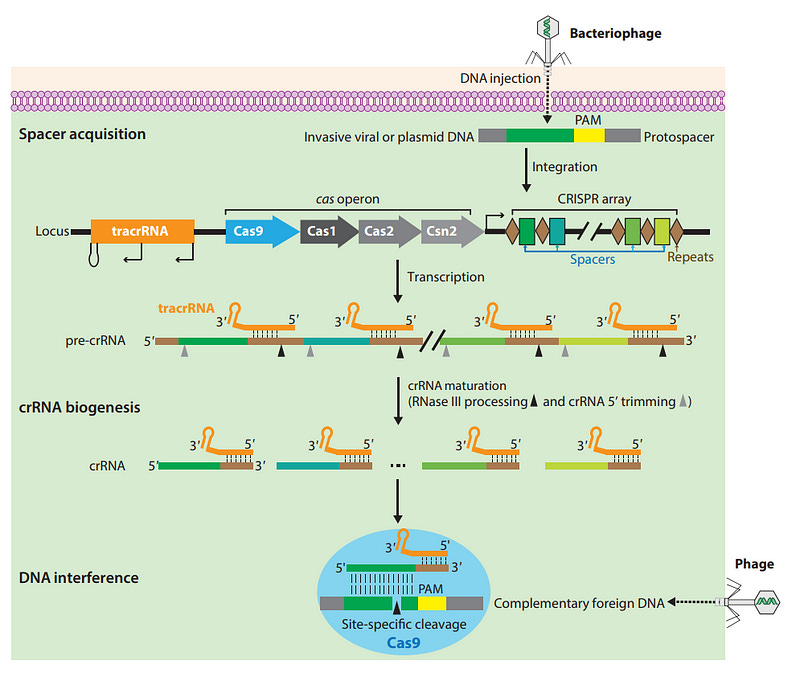
This is the biology behind the CRISPR-Cas system, which has now been adapted into a cutting-edge genetic engineering tool. Cas9, isolated from S. pyogenes, is loaded with Single Guide RNA (sgRNA). The sgRNA is a synthetic RNA molecule that combines both crRNA and tracrRNA. The sequence in this guide RNA can be modified to create specific cuts at desired locations in the genome.
After the DSB has been introduced at the target sequence, we can induce the cell for Non-Homologous End Joining (NHEJ) or Homology Dependent Repair (HDR) to either knock out a specific sequence/gene or introduce our desired sequences/gene at the DSB site.
Given the precision, efficiency, and ease of use of the CRISPR-Cas system for gene editing, it has been widely embraced by the academic and scientific community.
References:
Further Reading: