Terminologies Used:
RNA aptamers: Specific RNA sequences that can bind to target molecules (ligands) and form a binding pocket, allowing them to regulate gene expression.
Cell classifier: A specialized synthetic circuits that can distinguish different cell types.
Shine-Dalgarno Sequence: A prokaryotic ribosomal binding site sequence, important for translation initiation.
Kozak sequence: A eukaryotic ribosomal binding site sequence, important for translation initiation.
Cell-free system: A cell-free system is a collection of biological molecules that can be used to perform biological reactions in vitro, outside of a living cell.
IRES (Internal Ribosome Entry Site): A specific RNA structure that allows ribosomes to initiate translation internally within an mRNA molecule, bypassing the need for a 5' cap and the 5' cap-dependent translation initiation.
The functioning of electrical equipment, like a fan, can be simply controlled by an electrical switch, which basically regulates the flow of charges to the equipment. However, in a biological system — the cell — a switch, as well as the regulation that it confers and is needed in the system, is much more complex and versatile.
After the discovery of the Lac Operon, biologists started to uncover other regulatory networks and mechanisms of such regulation. Understanding the mechanism behind a biological function is often followed by attempts to engineer and modify it. Consequently, the scientific community began engineering synthetic switches that could be used to exogenously control the expression of transgenes [1].
Most of these switches were DNA-based, controlling expression at the transcriptional level. But recently, RNA switches or riboswitches have become popular as they are more convenient and less risky. Most RNA switches are basically RNA aptamers present in the 5’ UTR. These aptamers create a binding pocket for specific ligands. Depending on the presence or absence of specific ligands, these aptamers can either block or activate the translation of downstream genes, thus providing translational regulation.
However, these aptamer-based riboswitches require a trigger ligand. Administering trigger ligands to control the translation of RNA delivered into a cell is a good way to impart exogenous control. But for endogenous control, an RNA aptamer must be designed to be induced by endogenously present molecules, making it a tedious process. This is where toehold switches present an excellent RNA-only option.
Developed by Green et al. in 2014 [2], toehold switches can be easily employed as a cell classifier. They can be designed to sense specific endogenously produced small RNAs.
The ingenious yet simple design of a toehold switch contains a hairpin loop present at the 5’ end of an RNA molecule. This hairpin loop acts as a switch, and its structure is designed to specifically sequester the ribosome binding site (RBS) and start codon. There is a loop that contains the RBS, and the start codon forms a bulge in the middle of the hairpin loop (see fig. 1). The Watson-Crick base pair prevents the action of the ribosome, and translation is prevented.
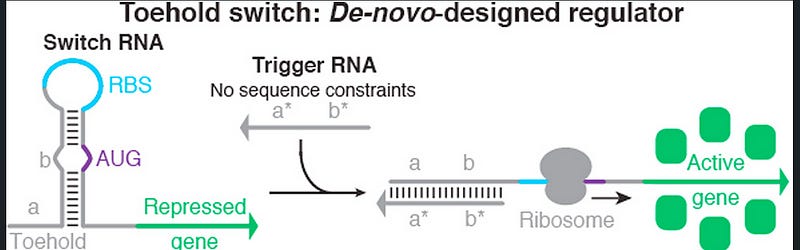
As seen in the figure, the left portion of the hairpin loop is called the toehold. This toehold domain can be made with any arbitrary sequence. An RNA molecule complementary to the toehold region can bind to the toehold. This causes an opening of the hairpin loop, which in turn makes the RBS and start codon accessible to the ribosome (see fig. 1). Hence, if a specific coding sequence is inserted downstream of this switch, it will be translated only in the presence of the trigger RNA. A short linker sequence is inserted between the coding sequence and the switch to provide structural flexibility and stability.
The toehold switch is, by default, in the off state. However, in 2019, a research group designed another switch based on toehold which is, by default, in the on state (see fig. 2) [3]. This again contained a similar design, but the base pairing in the lower stem is very weak. Thus, the ribosome is able to cut through the Watson-Crick bond, and hence drives the translation. But in the presence of a trigger RNA, the lower stem is sealed, and hence the structure resembles a normal toehold switch. So, in the presence of the trigger RNA, the translation is repressed.
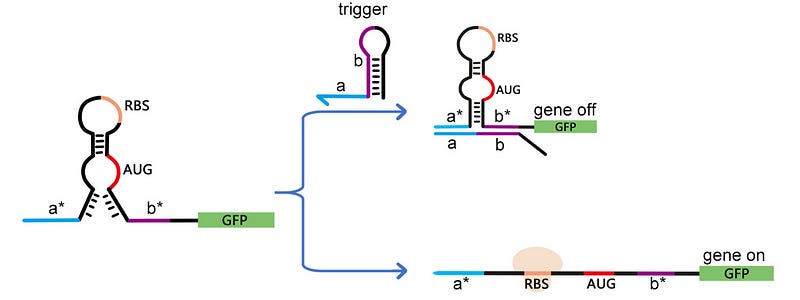
These switches were first designed for prokaryotic systems. Later, Wang et al. modified them for the mammalian system. Instead of an RBS (Shine-Dalgarno Sequence), the new switch contained the eukaryotic Kozak sequence in the loop, which was immediately followed by the start codon, which was this time base-paired to the left stem of the hairpin loop (see fig. 3). These mammalian toeholds could be activated by miRNA and hence can function as miRNA detectors [4].
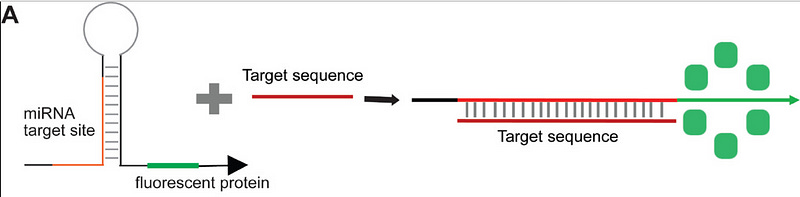
Wang et al., designed the first mammalian toeholds to detect miRNA-155 and miRNA-21. Since these are oncomirs, they suggested that these switches can be developed as diagnostics. Since then, multiple attempts have been made to utilize these switches to diagnose cancer as well as viral infections, including Covid [5][6]. Most of these diagnostics utilize a cell-free system. A coding sequence for the fluorescent protein is added downstream of the switch region. In the presence of trigger RNA, which acts as a diagnostic marker, fluorescence is reported.
Another research group designed a eukaryotic Toehold (or eToehold) in the year 2021. This toehold was more complex than the previous designs and contained an IRES. Based on the same principle, the IRES was disrupted using Watson-Crick base pairing. But the binding of a trigger RNA restores the function of IRES, thus permitting translation [7] (see fig. 4).
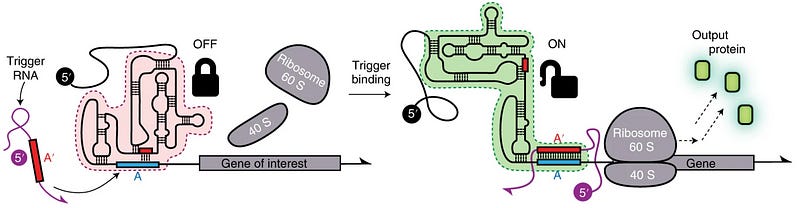
Despite a short history, Toehold switches seem to have a promising future. The ease with which they allow the possibility of controlling the expression of a gene both endogenously and exogenously is the main reason for their popularization. They can be easily designed with the help of software packages like NuPack [8]. Although prokaryotic switches have been used extensively, a eukaryotic version yet remains to be optimized.
References and Further Reading
Gene Switch: Novel Gene Switches for Targeted and Timed Expression of Proteins of Interest — ScienceDirect
Toehold Switch Design by Green et. al., 2014: Toehold Switches: De-Novo-Designed Regulators of Gene Expression — ScienceDirect
Translation Repressing Riboregulators (3WJ Repressors): De novo-designed translation-repressing riboregulators for multi-input cellular logic | Nature Chemical Biology
Mammalian Toehold: A Novel Synthetic Toehold Switch for MicroRNA Detection in Mammalian Cells | ACS Synthetic Biology
Covid Detection Using Toehold Switch: IJMS | Free Full-Text | Detection of Coronaviruses Using RNA Toehold Switch Sensors (mdpi.com)
Zika Virus Detection Using Toehold Switch: Rapid, Low-Cost Detection of Zika Virus Using Programmable Biomolecular Components — ScienceDirect
eToehold: RNA-responsive elements for eukaryotic translational control | Nature Biotechnology
NuPack Software Package: NUPACK